Understanding neuronal electrical activity is critical for anesthesiologists, particularly in how anesthetic agents influence cellular and systemic functions. Here, we review key physiological concepts: the resting membrane potential, action potentials, and refractory periods, which are crucial in anesthesia practice.
Resting Membrane Potential
The resting membrane potential is the voltage difference across a neuron’s cell membrane at rest, usually ranging from -60 to -90 millivolts (mV), with the inside of the cell being more negative relative to the outside. This potential is essential for the initiation of action potentials and is influenced by several factors:
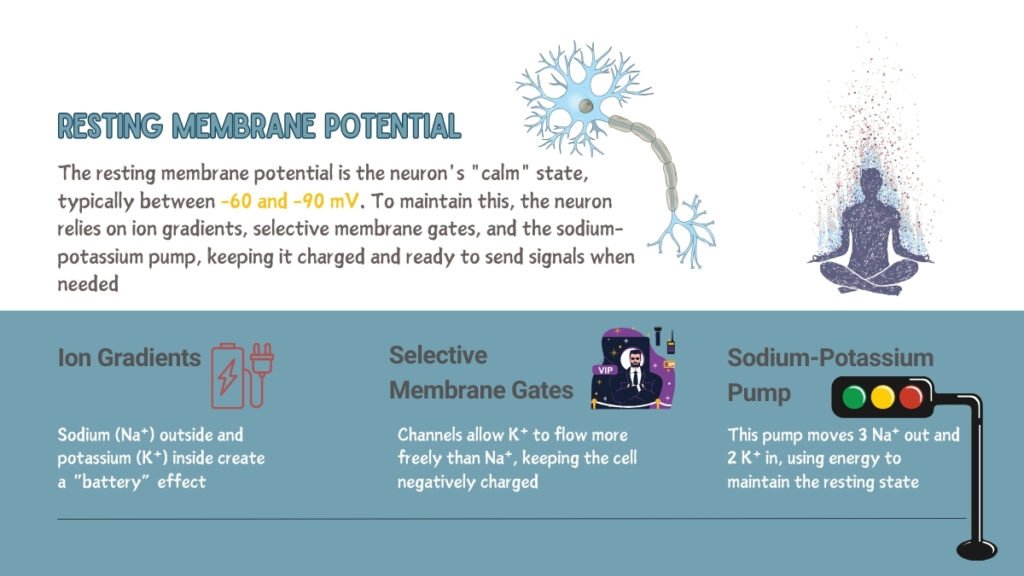
Ion Concentration Gradients
Sodium (Na⁺) ions are concentrated outside the cell, while potassium (K⁺) ions are in higher concentrations inside. This difference in concentration is fundamental for the voltage potential across the membrane. Neurons maintain this gradient using selective ion channels and active transport mechanisms, particularly the sodium-potassium pump, which creates an electrochemical gradient necessary for cellular excitability.
- Reference: Hille, B. (2001). Ion Channels of Excitable Membranes (3rd ed.). Sunderland, MA: Sinauer Associates. Hille describes the concentration gradients for Na⁺ and K⁺ and the cellular mechanisms that maintain them, forming the foundation of resting membrane potential.
Selective Membrane Permeability
The neuronal cell membrane selectively allows K⁺ ions to cross through leak channels, contributing to a more negative charge inside the cell. The Goldman-Hodgkin-Katz equation explains how varying permeabilities of Na⁺, K⁺, and other ions contribute to the overall membrane potential.
- Reference: Alberts, B., et al. (2014). Molecular Biology of the Cell (6th ed.). New York: Garland Science. This reference provides insights into selective ion permeability and how K⁺ leak channels help establish resting potential.
Active Transport (Sodium-Potassium Pump)
The Na⁺/K⁺ ATPase pump actively moves three Na⁺ ions out of the cell and two K⁺ ions into the cell against their concentration gradients, using ATP. This active transport is crucial in maintaining ionic imbalances necessary to sustain the resting potential.
- Reference: Kandel, E. R., Schwartz, J. H., & Jessell, T. M. (2000). Principles of Neural Science (4th ed.). New York: McGraw-Hill. This seminal text explains the role of the sodium-potassium pump in maintaining the ionic basis of resting membrane potential.
Action Potentials
An action potential is a rapid, temporary reversal in the membrane potential, facilitating neuronal communication and transmission of signals across tissues. This process occurs in distinct phases:
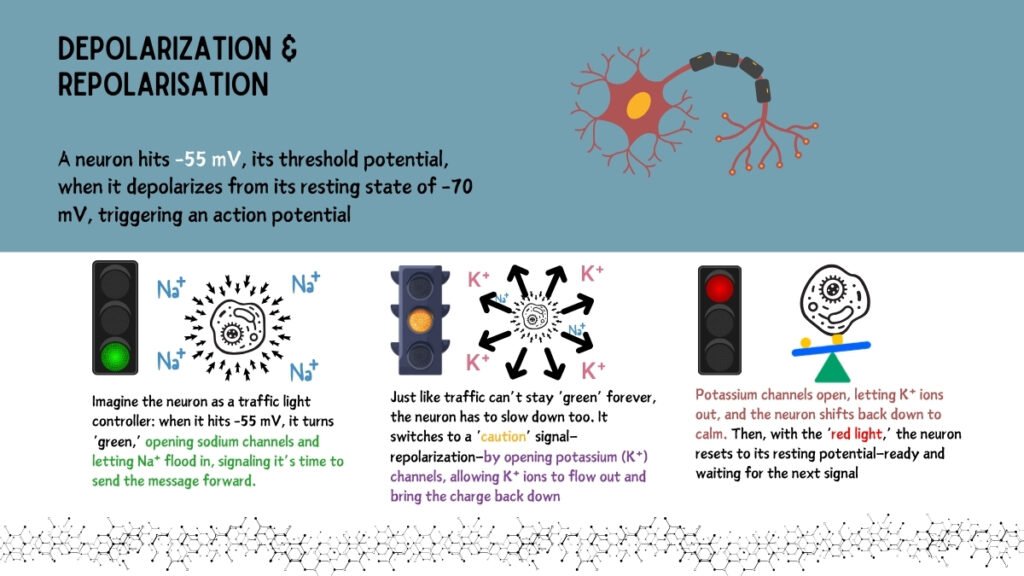
Depolarization
Upon reaching a threshold (~-55 mV), voltage-gated sodium channels open, allowing Na⁺ influx, which drives the cell’s interior to become more positively charged. This phase is critical in propagating the signal.
- Reference: Purves, D., et al. (2018). Neuroscience (6th ed.). Oxford University Press. This reference covers the stages of action potentials, detailing the role of Na⁺ in depolarization.
Repolarization
As the membrane potential peaks, sodium channels close, and potassium channels open, allowing K⁺ to exit the cell, which brings the potential back toward a negative value. This movement of K⁺ ions is essential for resetting the cell’s membrane potential.
- Reference: Levitan, I. B., & Kaczmarek, L. K. (2015). The Neuron: Cell and Molecular Biology (4th ed.). Oxford University Press. Detailed information on ion channel gating and repolarization is discussed in this text.
Hyperpolarization
Due to the delayed closure of K⁺ channels, the membrane potential becomes transiently more negative than the resting potential, resulting in hyperpolarization.
- Reference: Bear, M. F., Connors, B. W., & Paradiso, M. A. (2015). Neuroscience: Exploring the Brain (4th ed.). Wolters Kluwer Health. This resource explains hyperpolarization in the action potential sequence.
Return to Resting State
The Na⁺/K⁺ pump, along with K⁺ leak channels, reestablishes the original ion distribution and restores the resting membrane potential.
- Reference: Guyton, A. C., & Hall, J. E. (2020). Textbook of Medical Physiology (14th ed.). Elsevier. This text provides a detailed overview of ion pumps in maintaining resting potential after an action potential.
Refractory Periods
Following an action potential, the neuron enters a refractory period, preventing it from firing again immediately and allowing for controlled signaling:
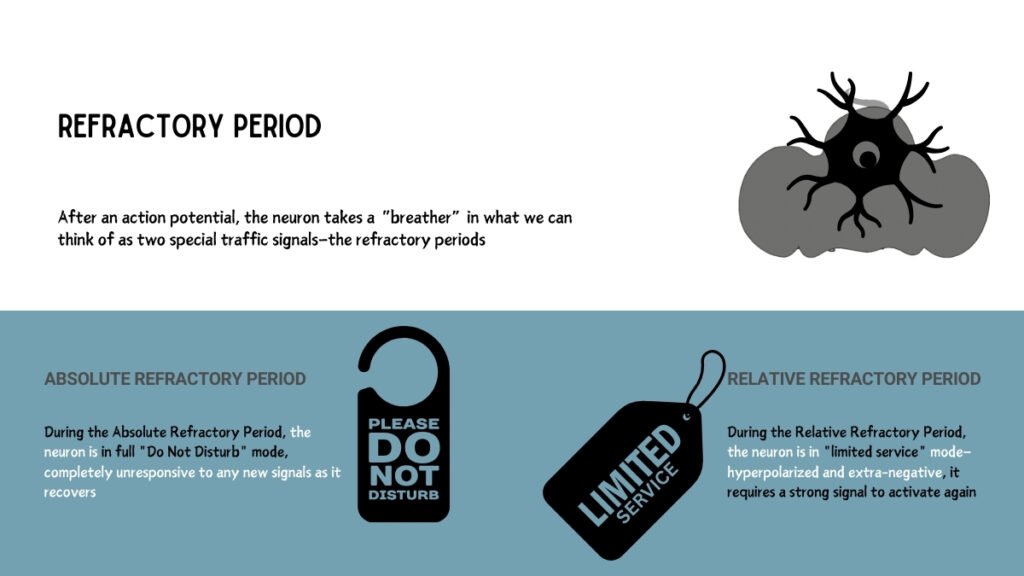
Absolute Refractory Period
During this period, sodium channels are inactivated, making it impossible for a second action potential to occur, regardless of stimulus strength. This ensures unidirectional propagation of the action potential.
- Reference: Johnston, D., & Wu, S. M. (1995). Foundations of Cellular Neurophysiology. MIT Press. This book details the importance of the absolute refractory period in action potential propagation.
Relative Refractory Period
After the absolute phase, a neuron requires a stronger-than-normal stimulus to generate another action potential, as it is in a temporarily hyperpolarized state.
- Reference: Nicholls, J. G., et al. (2012). From Neuron to Brain (5th ed.). Sinauer Associates. Nicholls et al. discuss both absolute and relative refractory periods and their role in neuronal signal transmission.
Relevance to Anesthesia
Anesthesia often involves modulating ion channels or influencing membrane potentials, essential in understanding and managing anesthetic agents effectively:
- Volatile Anesthetics (e.g., Isoflurane, Sevoflurane)
These agents modulate GABA-A receptors, increasing inhibitory effects and dampening excitatory responses via glutamate receptors, thereby affecting membrane potentials and neuronal excitability.
Reference: Hemmings, H. C., & Egan, T. D. (2019). Pharmacology and Physiology for Anesthesia (2nd ed.). Elsevier. Hemmings and Egan provide a comprehensive discussion on the impact of volatile anesthetics on neuronal excitability.
2. Local Anesthetics (e.g., Lidocaine)
Local anesthetics block voltage-gated sodium channels, preventing the initiation and propagation of action potentials, thus reducing sensory transmission and pain perception.
Reference: Miller, R. D., & Cohen, N. H. (2014). Miller’s Anesthesia (8th ed.). Elsevier. This authoritative text describes the mechanism of action of local anesthetics on sodium channels
3. Intravenous Anesthetics (e.g., Propofol)
Propofol enhances GABA-A receptor activity, hyperpolarizing neurons and reducing the probability of action potential initiation, thereby inducing sedation.
Reference: Stoelting, R. K., & Hillier, S. C. (2014). Pharmacology and Physiology in Anesthetic Practice (5th ed.). Lippincott Williams & Wilkins. Stoelting and Hillier discuss propofol’s effects on GABA-A receptors and its implications in anesthesia